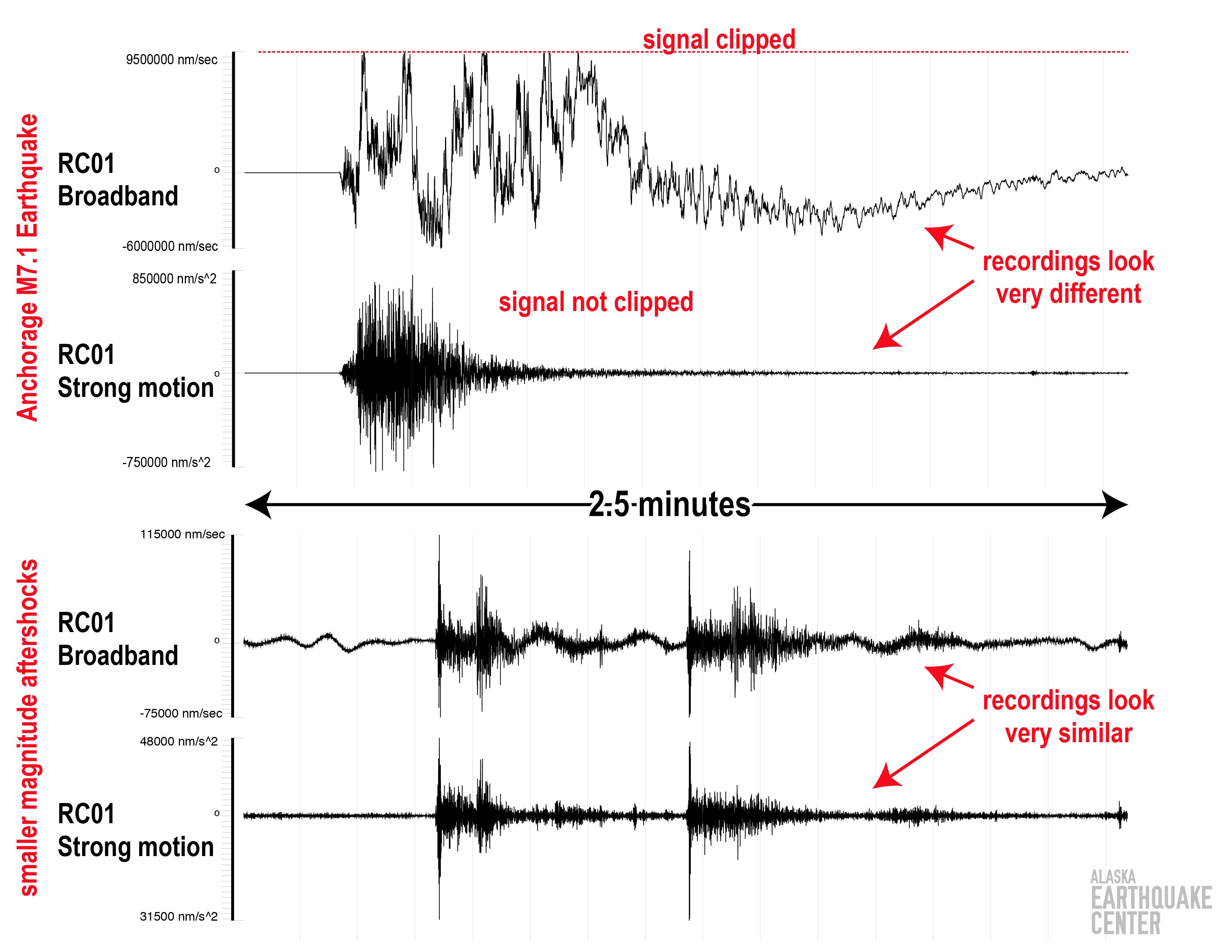
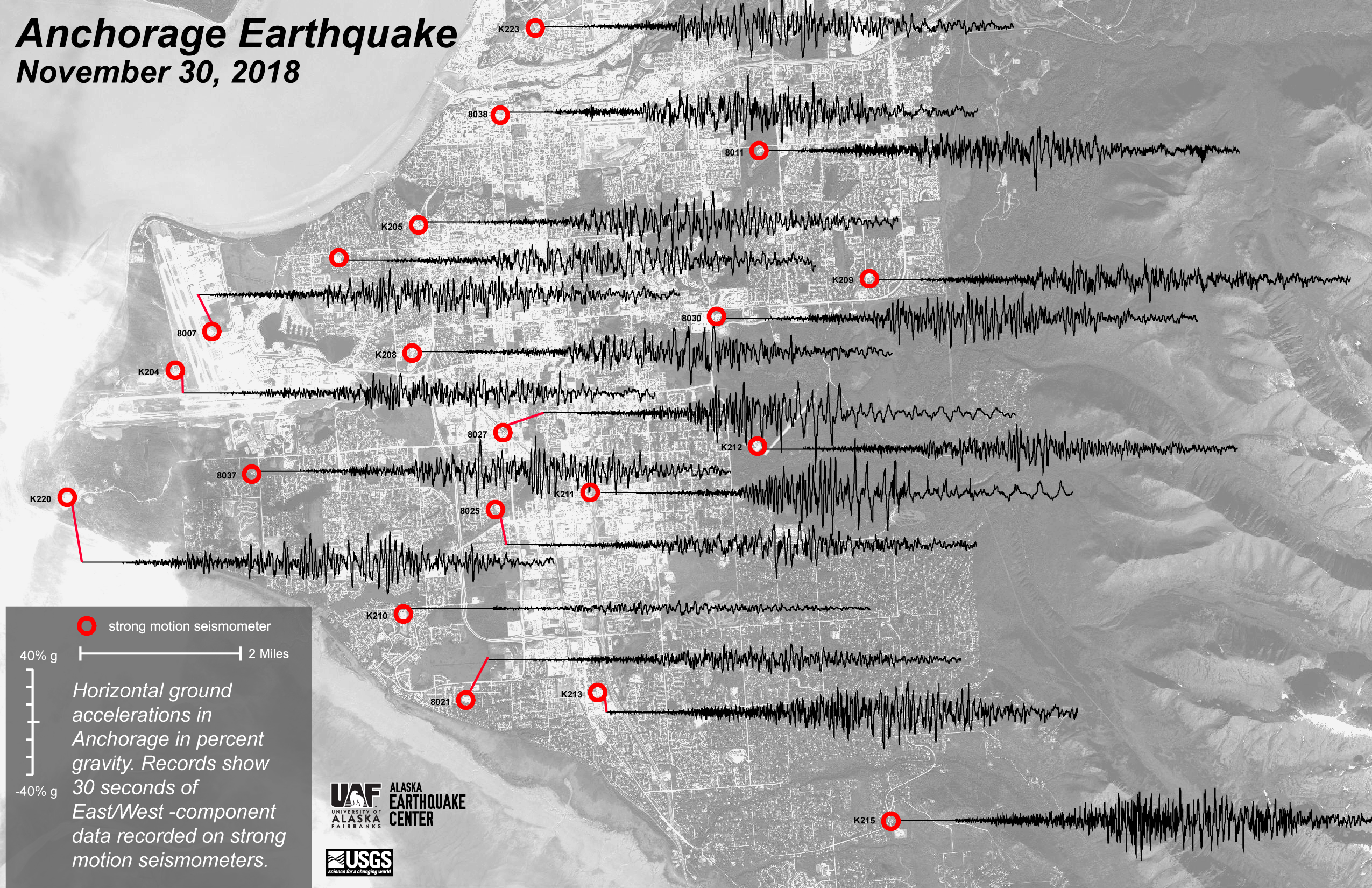
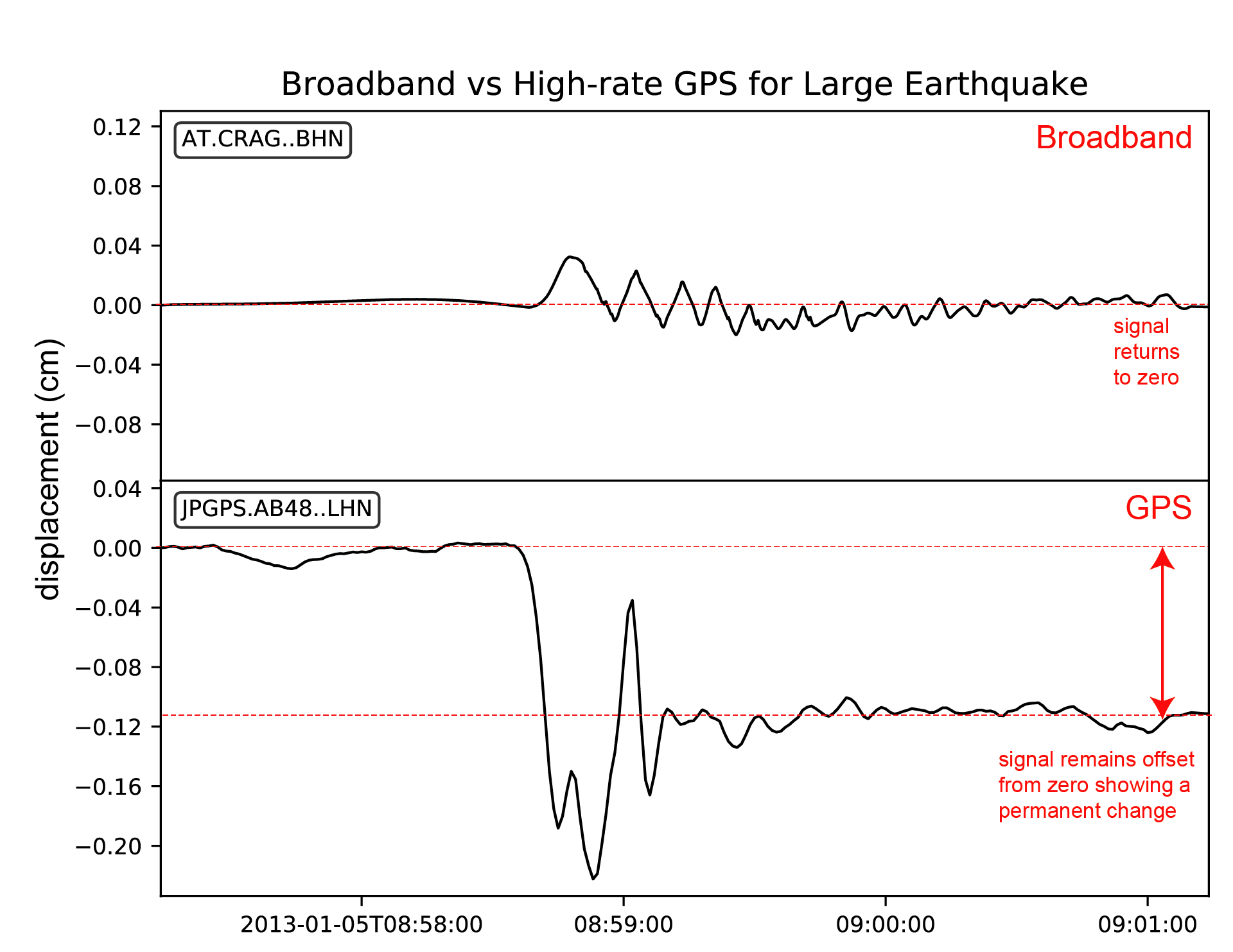
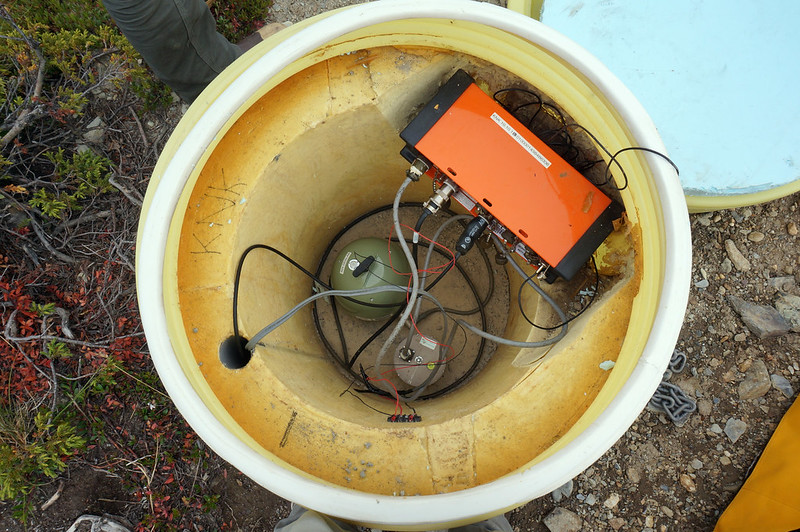
You may be surprised at the diversity of sensors that are able to detect seismic waves. Everything from the accelerometer in your cell phone to multi-million dollar satellites orbiting at altitudes of over 12,000 miles are capable of recording seismic vibrations. Surfers who visit the tsunami-prone Mentawai islands in Sumatra often invert an empty glass bottle on the floor before retiring, creating a very rudimentary ground-motion sensor in the absence of cell-phone coverage in that remote Indonesian region! This article will focus on three of the most ubiquitous seismic sensors in use today: broadband seismometers, strong-motion seismometers, and the Global Navigation Satellite System (GNSS).
Not all seismometers are created equal because not all ground motions are the same. When a seismic observatory such as the center records a ground motion, that recording may be processed in a variety of ways to achieve different purposes, and different types of seismometers excel at different tasks. The workhorse sensor for the center is the broadband seismometer. These extremely sensitive devices record seismic energy across a large range of frequencies (thus the “broadband” term). They usually record seismic velocity on three channels: up-down, east-west, and north-south. This allows them to capture the complexity of seismic waves as they move through the three-dimensional earth. These sensors excel at “listening” to the earth for weak motion—very small ground motions that are induced by small local or regional earthquakes, or very distant earthquakes. When the center’s website reports, for example, a magnitude 1.1 earthquake in the Brooks Range, that detection was made possible by our network of broadband seismometers. Broadbands are also very astute at recording seismic noise. This may not seem very useful, but this ambient, or background, noise is special. It is the “hum of the earth,” induced primarily by wave action at the coasts, and seismologists use it to study the geological structure of the rocky crust. Broadband seismometers form the backbone of the center’s monitoring capability.
From the above description, it may seem that broadbands are the only sensors that a seismologist would need. However, they do have a crucial limitation. Because they are optimized for recording faint, weak-motion signals, they do not do well in the large ground motions induced by strong or nearby earthquakes. Strong shaking will cause broadband seismometers to “clip”, or go off scale (see Figure 1). This phenomenon is similar to what happens if a stereo sound-system is too loud for its speakers, causing them to buzz and not properly reproduce the music. This is unfortunate, because it means that broadband sensors perform poorly, at least locally, at recording big earthquakes, and big earthquakes are the most societally impactful seismic events. After all, the definition of strong motion is ground motion that impacts people or their environment. Fortunately, the center operates another class of sensor called a strong-motion seismometer. As the name implies, while these do not excel at detecting the very faint seismic signals that are captured by broadbands, they have a large dynamic range, and can record even very large ground motions. This makes them extremely well-suited to engineering applications; dense networks of these sensors will often be located in urban environments such as Anchorage (see Figure 2) or around critical infrastructure such as power plants and the Trans Alaska Pipeline. In fact, although there is overlap, you can roughly think of broadbands seismometers as used for scientific purposes, while strong-motion sensors are for engineering.